IACC Strategic Plan
For Autism Spectrum Disorder
2016-2017
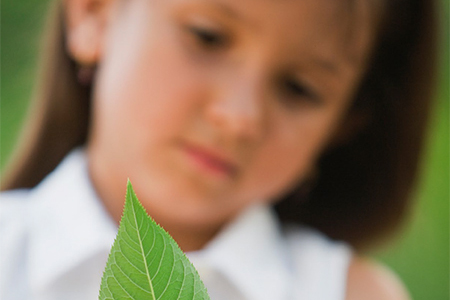
Introduction
Aspirational Goal: Causes of ASD will be discovered that inform diagnosis, prognosis, and interventions and lead to prevention or preemption of the challenges and disabilities of ASD.
Since the last Strategic Plan update published in 2013, there have been substantial advances in the understanding of factors that contribute to a diagnosis of autism spectrum disorder. Few would dispute that the causes of ASD are many and include both genetic and environmental factors. There has been an increased appreciation in the last five years of the incredible complexity and interplay of these factors in the development of autism. Indeed, modifications in more than 100 genes are now known to increase the probability of an autism diagnosis1,2 and very reasonable predictions are that 1,000 or more autism risk genes may ultimately be identified.1 A plethora of potential environmental challenges have also been associated with autism, although studies in this area have not undergone the same exponential progress as genomics research. There has also been increasing emphasis on events during pregnancy, such as influenza infection, as potential causes of neurodevelopmental disorders. But, these studies have raised the interesting issue that environmental risks affect different people differently. This is the so-called "genes by environment" interaction. More and more modern medical problems are linked to the combination of a particular genome and a particular life history of environmental exposure (the exposome).
The title of this chapter, which has been modified from the 2013 Strategic Plan Update, emphasizes the desire to understand the causes of the disabling aspects of autism spectrum disorder. These go beyond the core symptoms of deficits in social communication and the occurrence of restricted patterns of behavior or interests to include what are typically referred to as co-occurring symptoms. In many cases, progress on the causes of these co-occurring symptoms is ahead of that for the core symptoms of autism. There is a growing appreciation that the causes of these medical problems urgently need to be addressed. They may be due to biological factors that are also causal of autism, manifestations of autistic behavioral problems such as poor diet that may lead to medical issues, or medical access issues which lead to poorer medical care. Regardless of the causes, this is a clear gap in autism research and intervention and urgently needs additional research attention and efforts at resolution.
The title to this chapter has changed (from "What caused this to happen and can it be prevented?") because the neurodiversity movement has had a great impact on the IACC and on the premises of the Strategic Plan enterprise. It is fully appreciated now that some features of autism should not necessarily be targets for prevention. As discussed above, it is the most disabling features of autism that are now the major targets of prevention or preemption. Discussions of the causes of ASD always ultimately touch on efforts at prevention. In the hypothetical situation that a known cause of autism is identified, the question arises whether the cause should be eliminated thus preventing some cases of autism. If the discussion were related to cancer, the answer would be clear. But, in autism it is not. There is clearly an increased sensitivity to any procedure or practice that would be directed at preventing the totality of autism, and this is reflected in the emphasis of this chapter.
Genetic Risk Factors
The application of genomic studies has significantly advanced the understanding of genetic risk factors for ASD. Dozens of new ASD susceptibility genes have been identified over the past 5 years and genome-wide technologies have facilitated a molecular diagnosis in ~5-40% of ASD cases. This range in the rate of detection depends on the group of subjects examined and the technology used. Studies comparing individuals with ASD to typically developing individuals have indicated that as much as 7-8% of people with ASD carry either a large pathological DNA deletion or duplication.3,4,5,6 Earlier results from DNA sequencing studies have further confirmed the role of gene-disruptive mutations in ASD,7,8,9,10 and more recent data also support and add to these findings.11,12,13 The rates of de novo (new, spontaneous, non-inherited) likely gene-disruptive (LGD) and missense mutations (which result in amino acid changes) are significantly higher in individuals with ASD compared to their unaffected siblings (while the rate of mutations which do not alter amino acid sequence or biology does not differ).12 As much as 21% of autism diagnoses may be accounted for by de novo single-nucleotide variant (SNV) and insertion/deletion mutations.14 Additionally, inherited LGD mutations have been found to show a preferential transmission from mothers to sons, indicating another potential risk factor contributing to an estimated 8% of autism diagnoses.15 Recent whole genome sequencing studies of a large diverse ASD collection revealed a molecular basis in 11.2% of participants, including the finding that 7.2% carried copy number variations (CNVs; repeated sequences in the genome that vary between individuals) or chromosomal abnormalities,16 which further emphasize the need to use comprehensive genomic technologies.17 Overall, a consistent observation emerging from genomic studies is the vast genetic heterogeneity involved in ASD.18,19,20
Follow-up evaluation of individuals with ASD who have disruptive mutations to the same gene or genomic region has further illuminated features and patterns of behaviors (sub-phenotypes) linked to these genetically defined subgroups. Clinical characterization of cohorts with disruptive gene mutations has revealed real, but subtle, phenotypic patterns tied to particular genes. Patterns of behavior linked to sub-phenotypes can prove helpful for establishing guidelines of care for clinicians. While major advances have been made through the application of genomic technologies, gaps exist in our understanding of the contribution of regulatory and other genomic regions to ASD risk. Whole genome sequencing will begin to illuminate the role of non-gene coding regions of the genome.
Heritability
ASD is highly familial, so that siblings of children with autism are 10-20 times more likely to receive an ASD diagnosis themselves than non-siblings.21,22,23 Twin studies beginning in 197724 have provided significant evidence that ASD is strongly associated with genetic factors. All 13 twin studies on autism to date have found genetic and environmental contributions to autism, although the proportions of the two factors and interpretations have varied substantially. One research team,25 for example, concluded that over 50% of the risk for autism in identical twins could be explained by shared environmental factors, whereas genetic heritability accounted for 37%. This somewhat surprising finding—that environmental factors contribute more substantially than genetics—has been challenged by a more recent, large-scale twin study,26 which found that the largest contribution to autism liability comes from additive genetic effects. A recent meta-analysis concludes that the causes of autism are due to strong genetic effects, and that shared environmental influences are seen only if the most severe forms of autism are included.27 A recent survey of autism twin studies finds that concordance for monozygotic twins is roughly 45%, versus 16% for dizygotic twins.28 Thus, twin and family data suggest that genetic variation between people accounts for a very substantial portion of the liability to ASD at a population level. But, if autism had a completely genetic etiology, we would expect a much higher concordance (or shared ASD) rate in monozygotic twins; the lower rate may reflect, in part, that even monozygotic twins do not share an identical environment prenatally.
Genomic Architecture
The recent successes in ASD genetic studies have confirmed the importance of genetic risk factors. Similar to other common psychiatric disorders, the genomic architecture of ASD is complex, involving both common and rare forms of genetic variation,29 including common polygenic (multi-gene) variation, de novo variation, copy number variation, and inherited rare variation.11,12,15,19,30,31,32,33 Common polygenic variation may account for the greatest fraction of genetic influence, and approximately 20-50% of population liability. De novo variation accounts for less liability at a population level, but can have a very strong impact on the individuals who carry such variants.12,32 These data represent population risk; a crucial next step is integrating our understanding of rare variants of large effect with more common polygenic risk factors to more accurately predict ASD on an individual level.
Sex Differences
The overrepresentation of males among those diagnosed with ASD has been observed for decades.34,35,36 Overall, the male to female (m:f) ratio is approximately 4:1, but that ratio varies substantially based on IQ and other features of ascertainment.34,37,38 Specifically, in individuals with ASD and very low IQ, the male:female ratio is commonly estimated at 2:1 or 3:1; in individuals with ASD and high IQ, the m:f ratio becomes very large, often 7:1 or greater. This pattern has been consistently observed during the period of otherwise rapid changes in the epidemiology of ASD.
There are several theories as to why males and females might differ in their observed ASD liability.36 Among biological theories, the extreme male brain hypothesis posits that the male brain is predisposed to many features that are on the ASD spectrum.39,40 The ‘female protective effect’ (FPE) hypothesis in ASD is amongst the most commonly investigated in recent genomics studies.41 The FPE hypothesis suggests that females are 'protected' from ASD (for unspecified reasons) such that, on average, a greater number of risk factors (or genetic "hits") is necessary for a girl to gain a diagnosis of ASD. In the context of de novo and gene-disruptive inherited variation, that suggestion has been supported by the recent genetics literature.6,12,15,42 Deleterious CNVs are three times more likely to be identified in autistic females when compared to males,42 and loss-of-function mutations show a maternal transmission disequilibrium suggesting mothers are more likely to be carriers of such mutations than fathers.15 Similarly, classes of de novo variation that are strongly associated with ASD risk are approximately twice as likely to be observed in female cases, as compared to male cases. Recent gene expression analysis demonstrates that autism risk genes, rather than being sexually dimorphic themselves, interact with pathways and cell types that themselves are sexually dimorphic.43
Overlap with Other Disorders
Neuropsychiatric and developmental disorders share many genetic risk factors, and this varies depending on the specific disorders being compared.30,44,45 ASD shares common risk genes with other neuropsychiatric disorders such as schizophrenia,46,47 and autism is sometimes a feature of other neurodevelopmental syndromes such as Fragile X syndrome, Rett syndrome, tuberous sclerosis, and Phelan McDermid syndrome.48,49,50,51 The common, polygenic influences on ASD risk are similarly associated with multiple phenotypic outcomes (different combinations of risk genes can lead to different neuropsychiatric and developmental conditions). In recent studies, and in contrast to the de novo findings, common polygenic risk for ASD has been positively associated with general cognitive ability, logical memory, and verbal intelligence.52 On average, ASD shares most risk with schizophrenia in the population, followed by bipolar disorder, but very little with substance abuse or major depression. It does not appear that this overlap involves the majority of common genetic risk for each disorder, and the extent to which overlap occurs, and what biological factors it represents, remain under investigation.
Rare familial mutations that cause syndromic ASD and de novo (spontaneous) genetic influences on ASD risk are also strongly associated with intellectual disability, epilepsy, and global developmental delay;12,53 neurological co-occurring conditions are identified in the majority of children with ASD.54,55 Some of the ASD-associated de novo events that result in problems in protein production are more likely to be seen in cases of intellectual disability than in ASD itself.56 Similarly, several CNVs that increase risk for schizophrenia also increase risk for ASD.57,58,59,60,61 This is consistent with these mutations having major effects on brain development, which subsequently can manifest as different clinical outcomes. However, there are many intellectual disability genes that do not appear to increase risk for ASD.62 So, understanding why some large-effect mutations that cause intellectual disability substantially increase risk for ASD, while others may not, remains an area of future investigation.63
Genetic Testing and Communication of Risk
Genetic testing is recommended by the Accreditation Council for Graduate Medical Education for those at increased risk for ASD.64,65 This includes chromosomal microarray (CMA) followed by Fragile X testing and other specific tests depending on the symptoms that are observed. Several studies review the current recommendations for genetic testing in ASD.55 In addition to its usage in research studies, whole exome sequencing (WES) has been shown to have a high yield in clinical populations with developmental disorders including ASD. Thus, we expect that WES will gradually supplant CMA.66,67
Given the incomplete penetrance of many large effect or familial genetic risk factors, care must be taken in pre-symptomatic or prospective risk counseling. Further understanding of the causal relationship between identified ASD risk genes and clinical outcomes is needed before guidelines for genetic counseling can be illuminated. Understanding parental concerns and attitudes when communicating complex genetic information that has an impact on family planning is also important.68,69
New Large-Scale Genetics Studies Launched in the Last 5 Years
Studies of the genetic architecture of ASD have resulted in the appreciation that much larger groups of subjects are needed to fully understand its complexity. In the last 5 years, several large-scale projects have been initiated. Recent large-scale efforts include MSSNG (funded by Autism Speaks), which seeks to sequence the genomes of 10,000 individuals affected by ASD, and the SPARK study (funded by the Simons Foundation), which seeks to sequence exomes of 50,000 families affected by autism. These studies are expected to not only identify additional autism risk genes but to also contribute to an understanding of the common variant patterns that enable expression of the mutations.
Policy Implications of Advances in Genomic Science
New technology and testing can also lead to increases in healthcare disparities; we must be vigilant to avoid this and support policies that enable access to all. Because of differences in population histories, understanding of genetic risk in one population may not be informative in others. This imparts an imperative to study diverse populations. Further, given the role of rare variants that will have very distinct frequencies in different populations, having information from diverse populations will be critical for the interpretation of genetic studies. When predictive testing is performed, care must be taken to ensure accurate prospective/predictive testing and that information about accurate probabilities of particular outcomes are communicated effectively and not mistakenly understood as absolutes. This requires genetic counselors or other professionals trained specifically in the communication of genetic risk to patients. This will be an increasingly important manpower issue as genetic information expands over the next decade.
Environmental Risk Factors, Individually and in Combinations Over Time
The growing number of studies that are exploring environmental risk factors reflect an emerging consensus that non-genetic avenues of research are also likely to bear fruit. In this Strategic Plan, it is advantageous for the IACC to adopt a broad definition of studies on "environment" as encompassing research on all potentially non-heritable etiologic influences. This includes studies of exogenous exposures such as pesticides, endocrine disrupting and other industrial chemicals, pharmaceuticals, heavy metals, infectious agents, dietary factors, as well as other factors, such as parental age, maternal medical conditions, birth complications, and time between pregnancies. Some of these "environmental" factors might themselves be genetically influenced, while others might be mediating the effects of exogenous exposure.
Prevention or Amelioration of Disabling Aspects of ASD
Research on environmental contributors to ASD should routinely collect and make use of data on specific ASD symptoms, the levels of symptoms and impairment, as well as co-occurring conditions. As linkages between exposures and specific impairing aspects of ASD are revealed, public health strategies can be tailored to prevent or mitigate these features by reducing harmful exposures and/or increasing factors that confer protection or resilience. Additionally, improved understanding of what role environmental factors play in ASD severity (including risk for co-occurring conditions) might eventually inform strategies for identifying children in need of specific types of early intervention services.
Susceptible Periods During Development
The concept of windows of susceptibility, a central principle in environmental health sciences, is relevant to studies of environmental risks in ASD. Many lines of evidence point to prenatal origins of ASD.70,71,72,73,74,75 In addition, very large epidemiological studies link maternal bacterial or viral infection during specific times of pregnancy to increased risk for ASD in the offspring.76
The periods of prenatal development that are most relevant to environmental risks for ASD are incompletely understood, however, and may be dosage- and/or exposure-dependent.77 When considered together, many existing ASD studies suggest that preconception and early gestation are vulnerable periods for environmental exposures. This has been supported by previous reports linking autism symptoms to maternal ingestion of drugs such as thalidomide78,79 and valproic acid.79,80 Other factors associated with ASD risk include preterm birth,81,82,83,84 advanced maternal and paternal age at conception85,86,87,88 and short inter-pregnancy interval.89,90 In addition, the preconception/periconception period may be critical for the observed association of decreased ASD risk with maternal folate intake.91,92
A few of the studies on air pollution exposure suggest an enhanced risk in the later part of pregnancy.93,94 Evidence from the broader neurotoxicology literature95also indicates that exposures in the late prenatal and early postnatal periods can exert significant effects on a wide range of brain and behavior phenotypes during the first years of life. All of these time windows cover critical stages of rapid brain development and are also characterized by immaturity of both the immune system and metabolic detoxification mechanisms. These features combine to offer vulnerability and provide biological plausibility for environmental impact on ASD risk extending from preconception into the early postnatal period. Additional attention to the timing of exposures relative to the cascade of events that unfold during brain development is needed to identify and understand the molecular basis of exposure-associated ASD risk. With this in mind, study designs and biomarkers of exposure should be chosen to capture prenatal and early life exposures.
Studies in Large and Diverse Populations
While the number of ASD epidemiology studies and the resulting data are growing through efforts such as the Early Autism Risk Longitudinal Investigation (EARLI) and Markers of Autism Risk in Babies-Learning Early Signs (MARBLES), most potential environmental risk factors have not been investigated sufficiently to draw firm conclusions.96 The limitations inherent to observational studies mean that multiple studies in different populations and settings, with high-quality measures of exposure and adequate controls, are needed to reconcile disparate findings and establish robust linkages of an environmental exposure to ASD risk. The likelihood that many different factors, each with modest effect, will contribute to ASD means that large sample sizes may be needed to detect associations with exposure, especially for those exposures with low prevalence.
Under-represented minority communities and low-income communities often face disproportionate exposure to harmful environmental chemicals;97,98,99 additional attention is needed to ensure that these populations are represented in ASD research and that disparities in environmental risk factor exposure are addressed. Inclusion of these vulnerable population subgroups in ASD studies may, in some regions, be particularly challenging when studies recruit from young children with a past ASD diagnosis. Data from the Autism and Developmental Disabilities Monitoring (ADDM) Network indicate that non-Hispanic black (NHB) children are significantly less likely than non-Hispanic white (NHW) children to receive an early comprehensive developmental evaluation,100 and Hispanic children are less likely to receive an ASD identification by age 8 in comparison to NHW and NHB children. These findings underscore the need to carefully consider case ascertainment strategies that do not rely solely on previous ASD diagnosis in designing studies of ASD risk factors.
Given the clear differential in ASD risk in males and females, studies which examine risk and protective factors within sex-specific subgroups are especially important. However, given the lower ASD prevalence in females, nearly all studies to date have not had a sufficient sample of females to conduct such analyses. Thus, additional efforts are needed to increase representation of females in ASD studies to enable meaningful analyses of sex-specific differences and the role of both genetic and environmental factors in affecting those differences. The Environmental Influences on Children’s Health Outcomes (ECHO) initiative of the National Institutes of Health is combining data from more than 78 cohorts comprising approximately 50,000 children and 40,000 women. Although the extent of ASD-related measures that are, or will be, included in ECHO has not yet been established, this initiative represents an exceptional opportunity to study ASD-related traits in large and diverse populations.
Exposure Science
One of the most significant obstacles facing epidemiologic studies of environmental risks for ASD is exposure assessment. In many studies, exposure measures are not readily available for very early developmental periods and rely on indirect methods (e.g., participant recall of prior exposures), or utilize one or two biologic measurements of compounds with very short half-lives. Direct exposure assessment, such as through personal monitoring or use of an adequate time-course of exposure biomarkers, is expensive and burdensome for participants. Consequently, deep characterization of exposure during etiologically relevant time periods is typically limited to studies with small numbers of participants, yielding low power.
The recent development and application of the concept of the "exposome"101,102 represents a key advance that could accelerate progress in identifying environmental risks for ASD. The concept of the exposome calls attention to the totality of exposures across an individual’s lifespan.103,104 In addition to the universe of external environmental factors, the exposome concept can be extended to include endogenous biomarkers of exposure response – internal exposures that originate from metabolism and other cellular processes – as well as more general external factors that constitute social determinants of health. Measuring the exposome comes with challenges in capturing and integrating many individual measures over time. Recognizing that no single approach or tool likely will suffice, the field is embracing a multifaceted strategy, using multiple tools to help characterize the exposome. For example, use of personal sensors and mobile devices can be harnessed to capture many aspects of the exposome in real time. Refinement of more targeted, conventional exposure assessment tools also has a place in characterizing the exposome.
General "omics" approaches such as transcriptomics, proteomics, metabolomics, and epigenomics show promise in identifying molecular response profiles that can be linked to exposures,105,106,107 and in some cases, these profiles persist over time. These downstream biomarkers may suggest groupings of exposures that operate by similar pathways. Linking direct measures of either individual or classes of exposures or the broad exposome with early "omic" markers of biological response in both targeted and non-targeted data analyses can provide complementary information of potential etiologic relevance.
Because exposomic approaches have the potential to generate high-dimensional exposure data, discovery-based analytic methods analogous to those being used in genomics can potentially be applied to uncover novel environmental risk factors – ones that would be missed by approaches that focus on a small number of established or suspected neurotoxicants. An exposomic approach also is well-suited to the simultaneous consideration of multiple exposures and risk factors; although, as the genomics field has learned, very large samples are needed to achieve significance in these kinds of analyses.
Linkages Between Genes And Environment
Gene and Environmental Studies
Despite general agreement that both environment and genetics contribute to ASD risk, only modest progress has been made in identifying gene-environment interactions. A few epidemiology studies, such as the Childhood Autism Risk from Genes and Environment (CHARGE) study, have reported an interaction of exposures with common or rare structural genetic variation.92,108,109,110,111 However, each study focused on different combinations of exposures and genes, they lack independent replication, and two of these studies addressed severity of symptoms rather than impacts on incidence.108,111 Many large ASD genetic collections have been assembled but most include minimal or no exposure information. On the other hand, studies focused on environmental risks often feature deep exposure assessment and have incorporated some genetic information, but smaller sample sizes constrain the power of gene-environment interaction analyses.
A major gap has been identified and a concerted effort is needed to enrich existing, ongoing ASD studies by adding genetic data collection to environmental studies and exposure measures to genetic studies. Identifying and accounting for genetically driven effects on exposure levels is critical for interpretation of ASD-exposure associations. In the autism literature, it is notable that the genes implicated in the folic acid association with decreased ASD risk have not emerged in any of the genetic studies using genome-wide screening. Only in mothers who had low folic acid intake do those one-carbon metabolizing genes appear to play an etiologic role.109 At this stage, it appears that the presence of a ‘main effect’ (a risk factor not dependent on another factor) is not always detectable and that investigation of interacting factors should not require that a main effect of a specific environmental factor or gene/SNP be known.
Availability of low-burden exposure measures that can be incorporated in large-scale genetic studies, perhaps leveraging innovations in exposomics or epigenomics, is a high priority. Once these data exist in concert in large sample sets, new statistical and analytic approaches for gene-environment discovery in human population research can be applied.112 Polygenic risk scores have seen increasing use in complex disease studies and can yield improved efficiency for detecting interaction of genetic risk with candidate environmental exposures. The construction of a "polyenvironment" score, analogous to a polygenic risk score, could be explored to summarize information from several exposures thought to be acting through common mechanisms for use in genetic/genomic studies. Other approaches might include measures of genomic instability such as global copy number burden, used in two different gene-environment interaction studies.111,113
Mechanisms of Environmental Risk and Gene-Environment Interaction
Increasing knowledge of genetics has led scientists to understand gene pathways that affect neural circuits rather than single genes acting in isolation. Early studies have demonstrated the convergence of genetic influences and environmental factors in the activity of these different gene pathways,114 providing evidence that genes and the environment might work synergistically, rather than additively. Studies that move beyond identification of genetic and environmental risk factors to reveal functional biological consequences associated with these risk factors are a priority. Epigenomics, metabolomics, transcriptomics, and proteomics can provide useful functional readouts for this purpose.
Model systems provide an attractive means for supporting causality and understanding biological mechanisms that underlie associations observed in human studies. Human induced pluripotent stem cells (hiPSCs) generated from individuals affected by ASD with a known genetic background are being used increasingly to study ASD.115 These provide a unique opportunity to assess susceptibility of early developmental processes to environmental chemicals in the context of defined genetic risk.116 There are a few reports of screening or computational approaches used to identify possible environmental exposures that could be priorities for pursuit in human studies.114,117 The Collaborative Cross and Diversity Outbred mouse populations118 represent important mouse resources that could be harnessed to dissect the contribution of environment to complex disorders such as ASD. Additional efforts that bring together interdisciplinary teams to facilitate integrative analyses and bidirectional flow of clues from human observational studies to laboratory-based experiments in model systems are warranted.
In addition to the utility of epigenomics (the study of the complete set of epigenetic modifications – such as methylation – on the genetic material of a cell) as easily attainable exposure biomarkers, many researchers recognize the potential for mechanistic roles in ASD. Epigenomics is a leading candidate for mediating effects of exposures on regulation of transcription (the first step in gene expression)119,120,121 and could provide a point of convergence for genes and environment in autism risk. Multiple lines of evidence implicate altered epigenetic marks in ASD etiology. Several known genetic disorders with ASD-related presentation, such as Fragile X and Angelman syndrome, have established epigenetic mechanisms. Further, results from rare-variant ASD genetic discoveries point to remodeling of genetic material as a shared pathway in ASD genetic risk. Few studies have directly examined chromatin marks or DNA methylation for association with ASD, but some small studies have observed associations.105,122,123
A significant body of work demonstrates that environmental chemicals can alter DNA methylation, and these alterations have been linked to changes in gene expression and a range of behavioral phenotypes.124,125,126 ASD studies that integrate methylation, exposure, and phenotype data in the same population are a priority. Research to establish whether epigenetic marks measured in peripheral tissues are predictive of changes in target tissues is especially important for interpretation of human studies.127 Also needed are studies that identify exposure-induced impacts on a full range of epigenomic mechanisms and that determine their relevance to ASD. Finally, research to understand how exposure-induced epigenomic changes may transmit autism risk across generations is warranted.
Multivariate Risk Across Complex Systems
There is a need to capitalize on findings emerging from existing studies to examine how genetic and environmental factors interact to contribute to phenotype, not only at the molecular and cellular level, but also in the broader physiological context. For example, a substantial body of work implicates immune dysregulation in ASD, including the association of ASD with maternal infections and autoantibodies, cytokine and other immune biomarker signatures, functional alterations in immune cell subsets,128 and differential expression of innate immune and inflammatory genes.129 These findings together have motivated studies exploring how a range of environmental exposures may contribute to the immune alterations observed in ASD, some of which have been detectable at birth.130
The endocrine system is another promising area of inquiry. The established role of hormonal systems in brain development, the marked male bias in ASD, and a growing recognition that many environmental chemicals act as endocrine disrupting chemicals (EDCs) sets the stage for investigations exploring possible links between ASD and EDCs.131 Some research studies have suggested that factors that protect females from autism risk may be found on hormone-sensitive genes,132 and these could be targets for EDCs. Further work elucidating connections across metabolic, hormonal, and central nervous systems in the context of EDCs is needed.
The microbiome (the combined genetic material of the microorganisms in the body) represents a third priority area of inquiry. There is increasing evidence for links between the gut microbiome, brain, and behavioral phenotypes relevant to ASD.133,134 The microbiome is also emerging as an important component of response to environmental exposure. Studies have demonstrated persistent changes in the function of the microbiome after exposure to immune activation and environmental chemicals,135,136 particularly during early life when the microbiome is being colonized. A role for the microbiome in metabolism of environmental chemicals is now established.137,138 This means that differences among individuals in microbiome composition can affect the internal dose and biotransformation of toxicants and act as susceptibility factors. Small clinical studies using antibiotics or microbiome transplant support a potential role for microbial imbalance in contributing to the cause of core ASD behaviors. Taken together, these data suggest possible linkages among exposures, microbiome function, and ASD phenotypes. This is an area that has only begun to receive research attention which should be expanded in the foreseeable future.
Resources to Accelerate Environmental Risk and Gene-Environment Research
Broad Data and Resource Sharing
As the number of studies focusing on environmental risks for ASD increases, attention to broad data access and sharing becomes critical for enabling reuse and extracting the maximal value from the data that have been collected. Consideration of privacy and consent issues in environmental health data is needed to ensure the development and implementation of policies that protect privacy while ensuring the value of shared data. Combining data across observational studies can yield increased power and strengthen generalizability, yet heterogeneity of the types of exposure measures used creates challenges for both meta-analyses and pooled analyses of primary data. On the other hand, when different types of measures of exposure in different studies all lead to consistent findings, that consistency alone increases confidence in the conclusions.
The development of consensus data standards will make it possible for investigators to consider, at the outset of a study, inclusion of common environmental measures/ standards.139 Use of low burden exposure measures, such as those available through PhenX140 or the Early Life Exposure Assessment Tool (ELEAT), enable genetics researchers to enrich their analyses to account for environmental contributions to risk. Increased sharing of study-specific exposure instruments and methods is another area of need. The National Database for Autism Research (NDAR) currently provides a robust platform for making data – particularly data in standard formats – easy-to-find and accessible. Implementing common data standards for exposures could facilitate the incorporation of these type of data in NDAR.
In addition, genetic databases including MSSNG and the Autism Sequencing Consortium (ASC) are anticipated to provide mechanisms for expanded data libraries to include key environmental variables, allowing for assessment of gene-environment interactions. Recent findings108,111 illustrate the benefits of incorporating environmental information in large data resources. With regards to mechanistic tools, new models of ASD, especially those with distinguishing genetic mutations of interest, should be made widely accessible to researchers. This can include, but is not limited to, sharing breeding pairs of animal models with commercial vendors for their widespread distribution. Finally, to realize the potential impact of data sharing, efforts must be put into the analytic approaches needed to make gene-environment discoveries from the aggregation or collective analysis of large heterogeneous data sources. Efforts that encourage methodological development as well as bioinformatics implementation and secondary data analysis funding will be necessary.
Interdisciplinary Training and Career Development
The workforce needs related to environmental research in ASD align with an increasing recognition that solving complex questions will require team science approaches. Programs and opportunities that train scientists and support research and networking programs in ways that encourage crosstalk and coordination of efforts spanning cellular and molecular neurobiology, toxicology, genetics, epidemiology, and exposure science are needed. Training opportunities should be created around novel statistical and big data approaches geared toward complex exposure data, with the goal of accelerating analyses that address multivariate risk.
Public Health Implications
Communication and Dissemination Activities for Environmental Risk Findings
The multivariate risk structure of ASD, with many factors contributing modest risks, and different combinations of risks likely to operate in different individuals with ASD, presents challenges for communicating findings to affected families and the broader public. Epidemiologic studies that report associations of specific exposures with ASD at the population level can lead to serious misinterpretation if extrapolated to individual cases, and a focus on individual risks can mask the importance of exposures whose modification could have substantive impact when measured across the population. Moreover, the limitations inherent to observational studies means that results of a single study require additional independent studies for replication and assessment of generalizability. Conflicting findings among studies are common, and may reflect spurious results or an unappreciated dependency of the association on other factors. Additionally, it is particularly difficult to separate the effects of some exposures from other factors, due to inherent collinearity – for example, distinguishing true medication effects from effects due to the underlying health condition for which medication was required. For these reasons, communicating environmental and genetic findings in ASD requires careful attention to context, including providing information about the strength of any newly reported finding on the scale most appropriate for the audience, the difference between cause and association, the specific potential limitations of any individual study including the possibility of unmeasured confounding, the population attributable risk, and the need for additional studies to confirm the association.
Summary
In many cases, risk factors for ASD are shared by other disorders, and additional research is needed to ensure that corresponding public health efforts will have broad utility for protecting health beyond the implications for autism. The hope of identifying and understanding ASD risk factors is that they can be mitigated to reduce ASD-related disability.
Objectives
OBJECTIVE 1: Strengthen understanding of genetic risk and resilience factors for ASD across the full diversity and heterogeneity of those with ASD, enabling development of strategies for reducing disability and co-occurring conditions in ASD.
Examples:
- Understand the contribution of regulatory and other genomic regions to ASD risk. Whole genome sequencing will begin to illuminate the role of non-gene coding regions of the genome.
- Identify additional autism risk genes but also contribute to an understanding of the common variant patterns that enable expression of the mutations.
- Understand the causal relationship between identified ASD risk genes and clinical outcomes so that guidelines for genetic counseling can be illuminated. Understand parental concerns and attitudes when communicating complex genetic information.
OBJECTIVE 2: Understand the effects on ASD risk and resilience of individual and multiple exposures in early development, enabling development of strategies for reducing disability and co-occurring conditions in ASD.
Examples:
- Understand the timing of exposures relative to the cascade of events that unfold during brain development to identify and understand the molecular basis of exposure-associated ASD risk.
- Conduct multiple studies in different populations and settings, with high-quality measures of exposure and adequate controls, to reconcile disparate findings and establish robust linkages of environmental exposure to ASD risk.
- Refine more targeted, conventional exposure assessment tools to characterize the exposome.
OBJECTIVE 3: Expand knowledge about how multiple environmental and genetic risk and resilience factors interact through specific biological mechanisms to manifest in ASD phenotypes.
Examples:
- Develop low-burden exposure measures that can be incorporated in large-scale genetic studies, perhaps leveraging innovations in exposomics or epigenomics.
- Move beyond identification of genetic and environmental risk factors to reveal functional biological consequences associated with these risk factors.
- Integrate methylation, exposure, and phenotype data in the same population.
References
1. De Rubeis S, Buxbaum JD. Genetics and genomics of autism spectrum disorder: embracing complexity. Hum Mol Genet. 2015 Oct 15;24(R1):R24-31. [PMID: 26188008]
2. Geschwind DH, State MW. Gene hunting in autism spectrum disorder: on the path to precision medicine. Lancet Neurol. 2015 Nov;14(11):1109-20. [PMID: 25891009]
3. Pinto D, Pagnamenta AT, Klei L, Anney R, Merico D, Regan R, Conroy J, Magalhaes TR, Correia C, Abrahams BS, Almeida J, Bacchelli E, Bader GD, Bailey AJ, Baird G, Battaglia A, Berney T, Bolshakova N, Bölte S, Bolton PF, Bourgeron T, Brennan S, Brian J, Bryson SE, Carson AR, Casallo G, Casey J, Chung BH, Cochrane L, Corsello C, Crawford EL, Crossett A, Cytrynbaum C, Dawson G, de Jonge M, Delorme R, Drmic I, Duketis E, Duque F, Estes A, Farrar P, Fernandez BA, Folstein SE, Fombonne E, Freitag CM, Gilbert J, Gillberg C, Glessner JT, Goldberg J, Green A, Green J, Guter SJ, Hakonarson H, Heron EA, Hill M, Holt R, Howe JL, Hughes G, Hus V, Igliozzi R, Kim C, Klauck SM, Kolevzon A, Korvatska O, Kustanovich V, Lajonchere CM, Lamb JA, Laskawiec M, Leboyer M, Le Couteur A, Leventhal BL, Lionel AC, Liu XQ, Lord C, Lotspeich L, Lund SC, Maestrini E, Mahoney W, Mantoulan C, Marshall CR, McConachie H, McDougle CJ, McGrath J, McMahon WM, Merikangas A, Migita O, Minshew NJ, Mirza GK, Munson J, Nelson SF, Noakes C, Noor A, Nygren G, Oliveira G, Papanikolaou K, Parr JR, Par-aton T, Pickles A, Pilorge M, Piven J, Ponting CP, Posey DJ, Poustka A, Poustka F, Prasad A, Raoussis J, Renshaw K, Rickaby J, Roberts W, Roeder K, Roge B, Rutter ML, Bierut LJ, Rice JP, Salt J, Sansom K, Sato D, Segurado R, Sequeira AF, Senman L, Shah N, Sheffield VC, Soorya L, Sousa I, Stein O, Sykes N, Stoppioni V, Strawbridge C, Tancredi R, Tansey K, Thiruvahindrapduram B, Thompson AP, Thomson S, Tryfon A, Tsiantis J, Van Engeland H, Vincent JB, Volkmar F, Wallace S, Wang K, Wang Z, Wassink TH, Webber C, Weksberg R, Wing K, Wittemeyer K, Wood S, Wu J, Yaspan BL, Zurawiecki D, Zwaigenbaum L, Buxbaum JD, Cantor RM, Cook EH, Coon H, Cuccaro ML, Devlin B, Ennis S, Gallagher L, Geschwind DH, Gill M, Haines JL, Hallmayer J, Miller J, Monaco AP, Nurnberger JI Jr, Paterson AD, Pericak-Vance MA, Schellenberg GD, Szatmari P, Vicente AM, Vieland VJ, Wijsman EM, Scherer SW, Sutcliffe JS, Betancur C. Functional impact of global rare copy number variation in autism spectrum disorders. Nature. 2010 Jul 15;466(7304):368-72. [PMID: 20531469]
4. Sanders SJ, Ercan-Sencicek AG, Hus V, Luo R, Murtha MT, Moreno-De-Luca D, Chu SH, Moreau MP, Gupta AR, Thomson SA, Mason CE, Bilguvar K, Celestino-Soper PB, Choi M, Crawford EL, Davis L, Wright NR, Dhodapkar RM, DiCola M, DiLullo NM, Fernandez TV, Fielding-Singh V, Fishman DO, Frahm S, Garagaloyan R, Goh GS, Kammela S, Klei L, Lowe JK, Lund SC, McGrew AD, Meyer KA, Moffat WJ, Murdoch JD, O'Roak BJ, Ober GT, Pottenger RS, Raubeson MJ, Song Y, Wang Q, Yaspan BL, Yu TW, Yurkiewicz IR, Beaudet AL, Cantor RM, Curland M, Grice DE, Günel M, Lifton RP, Mane SM, Martin DM, Shaw CA, Sheldon M, Tischfield JA, Walsh CA, Morrow EM, Ledbetter DH, Fombonne E, Lord C, Martin CL, Brooks AI, Sutcliffe JS, Cook EH Jr, Geschwind D, Roeder K, Devlin B, State MW. Multiple recurrent de novo CNVs, including duplications of the 7q11.23 Williams syndrome region, are strongly associated with autism. Neuron. 2011 Jun 9;70(5):863-85. [PMID: 21658581]
5. Sebat J, Lakshmi B, Malhotra D, Troge J, Lese-Martin C, Walsh T, Yamrom B, Yoon S, Krasnitz A, Kendall J, Leotta A, Pai D, Zhang R, Lee YH, Hicks J, Spence SJ, Lee AT, Puura K, Lehtimäki T, Ledbetter D, Gregersen PK, Bregman J, Sutcliffe JS, Jobanputra V, Chung W, Warburton D, King MC, Skuse D, Geschwind DH, Gilliam TC, Ye K, Wigler M. Strong association of de novo copy number mutations with autism. Science. 2007 Apr 20;316(5823):445-9. [PMID: 17363630]
6. Pinto D, Delaby E, Merico D, Barbosa M, Merikangas A, Klei L, Thiruvahindrapuram B, Xu X, Ziman R, Wang Z, Vorstman JA, Thompson A, Regan R, Pilorge M, Pellecchia G, Pagnamenta AT, Oliveira B, Marshall CR, Magalhaes TR, Lowe JK, Howe JL, Griswold AJ, Gilbert J, Duketis E, Dombroski BA, De Jonge MV, Cuccaro M, Crawford EL, Correia CT, Conroy J, Conceição IC, Chiocchetti AG, Casey JP, Cai G, Cabrol C, Bolshakova N, Bacchelli E, Anney R, Gallinger S, Cotterchio M, Casey G, Zwaigenbaum L, Wittemeyer K, Wing K, Wallace S, van Engeland H, Tryfon A, Thomson S, Soorya L, Rogé B, Roberts W, Poustka F, Mouga S, Minshew N, McInnes LA, McGrew SG, Lord C, Leboyer M, Le Couteur AS, Kolevzon A, Jiménez González P, Jacob S, Holt R, Guter S, Green J, Green A, Gillberg C, Fernandez BA, Duque F, Delorme R, Dawson G, Chaste P, Café C, Brennan S, Bourgeron T, Bolton PF, Bölte S, Bernier R, Baird G, Bailey AJ, Anagnostou E, Almeida J, Wijsman EM, Vieland VJ, Vicente AM, Schellenberg GD, Pericak-Vance M, Paterson AD, Parr JR, Oliveira G, Nurnberger JI, Monaco AP, Maestrini E, Klauck SM, Hakonarson H, Haines JL, Geschwind DH, Freitag CM, Folstein SE, Ennis S, Coon H, Battaglia A, Szatmari P, Sutcliffe JS, Hallmayer J, Gill M, Cook EH, Buxbaum JD, Devlin B, Gallagher L, Betancur C, Scherer SW. Convergence of genes and cellular pathways dysregulated in autism spectrum disorders. Am J Hum Genet. 2014 May 1;94(5):677-94. [PMID: 24768552]
7. O'Roak BJ, Vives L, Fu W, Egertson JD, Stanaway IB, Phelps IG, Carvill G, Kumar A, Lee C, Ankenman K, Munson J, Hiatt JB, Turner EH, Levy R, O'Day DR, Krumm N, Coe BP, Martin BK, Borenstein E, Nickerson DA, Mefford HC, Doherty D, Akey JM, Bernier R, Eichler EE, Shendure J. Multiplex targeted sequencing identifies recurrently mutated genes in autism spectrum disorders. Science. 2012 Dec 21;338(6114):1619-22. [PMID: 23160955]
8. O'Roak BJ, Vives L, Girirajan S, Karakoc E, Krumm N, Coe BP, Levy R, Ko A, Lee C, Smith JD, Turner EH, Stanaway IB, Vernot B, Malig M, Baker C, Reilly B, Akey JM, Borenstein E, Rieder MJ, Nickerson DA, Bernier R, Shendure J, Eichler EE. Sporadic autism exomes reveal a highly interconnected protein network of de novo mutations. Nature, 485(7397), 246-250. [PMID: 22495309]
9. Neale BM, Kou Y, Liu L, Ma'ayan A, Samocha KE, Sabo A, Lin CF, Stevens C, Wang LS, Makarov V, Polak P, Yoon S, Maguire J, Crawford EL, Campbell NG, Geller ET, Valladares O, Schafer C, Liu H, Zhao T, Cai G, Lihm J, Dannenfelser R, Jabado O, Peralta Z, Nagaswamy U, Muzny D, Reid JG, Newsham I, Wu Y, Lewis L, Han Y, Voight BF, Lim E, Rossin E, Kirby A, Flannick J, Fromer M, Shakir K, Fennell T, Garimella K, Banks E, Poplin R, Gabriel S, DePristo M, Wimbish JR, Boone BE, Levy SE, Betancur C, Sunyaev S, Boerwinkle E, Buxbaum JD, Cook EH Jr, Devlin B, Gibbs RA, Roeder K, Schellenberg GD, Sutcliffe JS, Daly MJ. Patterns and rates of exonic de novo mutations in autism spectrum disorders. Nature. 2012 Apr 4;485(7397):242-5. [PMID: 22495311]
10. Sanders SJ, Murtha MT, Gupta AR, Murdoch JD, Raubeson MJ, Willsey AJ, Ercan-Sencicek AG, DiLullo NM, Parikshak NN, Stein JL, Walker MF, Ober GT, Teran NA, Song Y, El-Fishawy P, Murtha RC, Choi M, Overton JD, Bjornson RD, Carriero NJ, Meyer KA, Bilguvar K, Mane SM, Sestan N, Lifton RP, Günel M, Roeder K, Geschwind DH, Devlin B, State MW. De novo mutations revealed by whole-exome sequencing are strongly associated with autism. Nature. 2012 Apr 4;485(7397):237-41. [PMID: 22495306]
11. De Rubeis S, He X, Goldberg AP, Poultney CS, Samocha K, Cicek AE, Kou Y, Liu L, Fromer M, Walker S, Singh T, Klei L, Kosmicki J, Shih-Chen F, Aleksic B, Biscaldi M, Bolton PF, Brownfeld JM, Cai J, Campbell NG, Carracedo A, Chahrour MH, Chiocchetti AG, Coon H, Crawford EL, Curran SR, Dawson G, Duketis E, Fernandez BA, Gallagher L, Geller E, Guter SJ, Hill RS, Ionita-Laza J, Jimenz Gonzalez P, Kilpinen H, Klauck SM, Kolevzon A, Lee I, Lei I, Lei J, Lehtimäki T, Lin CF, Ma'ayan A, Marshall CR, McInnes AL, Neale B, Owen MJ, Ozaki N, Parellada M, Parr JR, Purcell S, Puura K, Rajagopalan D, Rehnström K, Reichenberg A, Sabo A, Sachse M, Sanders SJ, Schafer C, Schulte-Rüther M, Skuse D, Stevens C, Szatmari P, Tammimies K, Valladares O, Voran A, Li-San W, Weiss LA, Willsey AJ, Yu TW, Yuen RK; DDD Study; Homozygosity Mapping Collaborative for Autism; UK10K Consortium, Cook EH, Freitag CM, Gill M, Hultman CM, Lehner T, Palotie A, Schellenberg GD, Sklar P, State MW, Sutcliffe JS, Walsh CA, Scherer SW, Zwick ME, Barett JC, Cutler DJ, Roeder K, Devlin B, Daly MJ, Buxbaum JD. Synaptic, transcriptional and chromatin genes disrupted in autism. Nature. 2014 Nov 13;515(7526):209-15. [PMID: 25363760]
12. Iossifov I, O'Roak BJ, Sanders SJ, Ronemus M, Krumm N, Levy D, Stessman HA, Witherspoon KT, Vives L, Patterson KE, Smith JD, Paeper B, Nickerson DA, Dea J, Dong S, Gonzalez LE, Mandell JD, Mane SM, Murtha MT, Sullivan CA, Walker MF, Waqar Z, Wei L, Willsey AJ, Yamrom B, Lee YH, Grabowska E, Dalkic E, Wang Z, Marks S, Andrews P, Leotta A, Kendall J, Hakker I, Rosenbaum J, Ma B, Rodgers L, Troge J, Narzisi G, Yoon S, Schatz MC, Ye K, McCombie WR, Shendure J, Eichler EE, State MW, Wigler M. The contribution of de novo coding mutations to autism spectrum disorder. Nature. 2014 Nov 13;515(7526):216-21. [PMID: 25363768]
13. Tammimies K, Marshall CR, Walker S, Kaur G, Thiruvahindrapuram B, Lionel AC, Yuen RK, Uddin M, Roberts W, Weksberg R, Woodbury-Smith M, Zwaigenbaum L, Anagnostou E, Wang Z, Wei J, Howe JL, Gazzellone MJ, Lau L, Sung WW, Whitten K, Vardy C, Crosbie V, Tsang B, D'Abate L, Tong WW, Luscombe S, Doyle T, Carter MT, Szatmari P, Stuckless S, Merico D, Stavropoulos DJ, Scherer SW, Fernandez BA. Molecular diagnostic yield of chromosomal microarray analysis and whole-exome sequencing in children with autism spectrum disorder. JAMA. 2015 Sep 1;314(9):895-903. [PMID: 26325558]
14. Ronemus M, Iossifov I, Levy D, Wigler M. The role of de novo mutations in the genetics of autism spectrum disorders. Nat Rev Genet. 2014 Feb;15(2):133-41. [PMID: 24430941]
15. Krumm N, Turner TN, Baker C, Vives L, Mohajeri K, Witherspoon K, Raja A, Coe BP, Stessman HA, He ZX, Leal SM, Bernier R, Eichler EE. Excess of rare, inherited truncating mutations in autism. Nat Genet. 2015 Jun;47(6):582-8. [PMID: 25961944]
16. C Yuen RK, Merico D, Bookman M, L Howe J, Thiruvahindrapuram B, Patel RV, Whitney J, Deflaux N, Bingham J, Wang Z, Pellecchia G, Buchanan JA, Walker S, Marshall CR, Uddin M, Zarrei M, Deneault E, D'Abate L, Chan AJ, Koyanagi S, Paton T, Pereira SL, Hoang N, Engchuan W, Higginbotham EJ, Ho K, Lamoureux S, Li W, MacDonald JR, Nalpathamkalam T, Sung WW, Tsoi FJ, Wei J, Xu L, Tasse AM, Kirby E, Van Etten W, Twigger S, Roberts W, Drmic I, Jilderda S, Modi BM, Kellam B, Szego M, Cytrynbaum C, Weksberg R, Zwaigenbaum L, Woodbury-Smith M, Brian J, Senman L, Iaboni A, Doyle-Thomas K, Thompson A, Chrysler C, Leef J, Savion-Lemieux T, Smith IM, Liu X, Nicolson R, Seifer V, Fedele A, Cook EH, Dager S, Estes A, Gallagher L, Malow BA, Parr JR, Spence SJ, Vorstman J, Frey BJ, Robinson JT, Strug LJ, Fernandez BA, Elsabbagh M, Carter MT, Hallmayer J, Knoppers BM, Anagnostou E, Szatmari P, Ring RH, Glazer D, Pletcher MT, Scherer SW. Whole genome sequencing resource identifies 18 new candidate genes for autism spectrum disorder. Nat Neurosci. 2017 Apr;20(4):602-611. [PMID: 28263302]
17. Talkowski ME, Rosenfeld JA, Blumenthal I, Pillalamarri V, Chiang C, Heilbut A, Ernst C, Hanscom C, Rossin E, Lindgren AM, Pereira S, Ruderfer D, Kirby A, Ripke S, Harris DJ, Lee JH, Ha K, Kim HG, Solomon BD, Gropman AL, Lucente D, Sims K, Ohsumi TK, Borowsky ML, Loranger S, Quade B, Lage K, Miles J, Wu BL, Shen Y, Neale B, Shaffer LG, Daly MJ, Morton CC, Gusella JF. Sequencing chromosomal abnormalities reveals neurodevelopmental loci that confer risk across diagnostic boundaries. Cell. 2012 Apr 27;149(3): 525-37. [PMID: 22521361]
18. Leppa VM, Kravitz SN, Martin CL, Andrieux J, Le Caignec C, Martin-Coignard D, DyBuncio C, Sanders SJ, Lowe JK, Cantor RM, Geschwind DH. Rare inherited and de novo CNVs reveal complex contributions to ASD risk in multiplex families. Am J Hum Genet. 2016 Sep 1;99(3):540-54. [PMID: 27569545]
19. Sanders SJ, He X, Willsey AJ, Ercan-Sencicek AG, Samocha KE, Cicek AE, Murtha MT, Bal VH, Bishop SL, Dong S, Goldberg AP, Jinlu C, Keaney JF 3rd, Klei L, Mandell JD, Moreno-De-Luca D, Poultney CS, Robinson EB, Smith L, Solli-Nowlan T, Su MY, Teran NA, Walker MF, Werling DM, Beaudet AL, Cantor RM, Fombonne E, Geschwind DH, Grice DE, Lord C, Lowe JK, Mane SM, Martin DM, Morrow EM, Talkowski ME, Sutcliffe JS, Walsh CA, Yu TW; Autism Sequencing Consortium, Ledbetter DH, Martin CL, Cook EH, Buxbaum JD, Daly MJ, Devlin B, Roeder K, State MW. Insights into autism spectrum disorder genomic architecture and biology from 71 risk loci. Neuron. 2015 Sep 23;87(6):1215-33. [PMID: 26402605]
20. Yuen RK, Thiruvahindrapuram B, Merico D, Walker S, Tammimies K, Hoang N, Chrysler C, Nalpathamkalam T, Pellecchia G, Liu Y, Gazzellone MJ, D'Abate L, Deneault E, Howe JL, Liu RS, Thompson A, Zarrei M, Uddin M, Marshall CR, Ring RH, Zwaigenbaum L, Ray PN, Weksberg R, Carter MT, Fernandez BA, Roberts W, Szatmari P, Scherer SW. Whole-genome sequencing of quartet families with autism spectrum disorder. Nat Med. 2015 Feb;21(2):185-91. [PMID: 25621899]
21. Constantino JN, Todorov A, Hilton C, Law P, Zhang Y, Molloy E, Fitzgerald R, Geschwind D. Autism recurrence in half siblings: strong support for genetic mechanisms of transmission in ASD. Mol Psychiatry. 2013 Feb;18(2):137-8. [PMID: 22371046]
22. Risch N, Hoffmann TJ, Anderson M, Croen LA, Grether JK, Windham GC. Familial recurrence of autism spectrum disorder: evaluating genetic and environmental contributions. Am J Psychiatry. 2014 Nov 1;171(11):1206-13. [PMID: 24969362]
23. Sandin S, Lichtenstein P, Kuja-Halkola R, Larsson H, Hultman CM, Reichenberg A. The familial risk of autism. JAMA. 2014 May 7;311(17):1770-7. [PMID: 24794370]
24. Folstein S, Rutter M. Infantile autism: a genetic study of 21 twin pairs. J Child Psychol Psychiatry. 1977 Sep;18(4):297-321. [PMID: 562353]
25. Hallmayer J, Cleveland S, Torres A, Phillips J, Cohen Lotspeich L, Croen LA, Ozonoff S, Lajonchere C, Grether JK, Risch N. Genetic heritability and shared environmental factors among twin pairs with autism. Arch Gen Psychiatry. 2011 Nov;68(11):1095-102. [PMID: 21727249]
26. Colvert E, Tick B, McEwen F, Stewart C, Curran SR, Woodhouse E, Gillan N, Hallett V, Lietz S, Garnett T, Ronald A, Plomin R, Rijsdijk F, Happé F, Bolton P. Heritability of autism spectrum disorder in a UK population-based twin sample. JAMA Psychiatry. 2015 May;72(5):415-23. [PMID: 25738232]
27. Tick B, Colvert E, McEwen F, Stewart C, Woodhouse E, Gillan N, Hallett V, Lietz S, Garnett T, Simonoff E, Ronald A, Bolton P, Happé F, Rijsdijk F. Autism spectrum disorders and other mental health problems: exploring etiological overlaps and phenotypic causal associations. J Am Acad Child Adolesc Psychiatry. 2016 Feb;55(2):106-13.e4. [PMID: 26802777]
28. Bourgeron T. Current knowledge on the genetics of autism and propositions for future research. C R Biol. 2016 Jul-Aug;339(7-8):300-7. [PMID: 27289453]
29. Geschwind DH, Flint J. Genetics and genomics of psychiatric disease. Science. 2015 Sep 25; 349(6255):1489-94. [PMID: 26404826]
30. Bulik-Sullivan B, Finucane HK, Anttila V, Gusev A, Day FR, Loh PR; ReproGen Consortium; Psychiatric Genomics Consortium; Genetic Consortium for Anorexia Nervosa of the Wellcome Trust Case Control Consortium 3, Duncan L, Perry JR, Patterson N, Robinson EB, Daly MJ, Price AL, Neale BM. An atlas of genetic correlations across human diseases and traits. Nat Genet. 2015 Nov;47(11):1236-41. [PMID: 26414676]
31. Bulik-Sullivan BK, Loh PR, Finucane HK, Ripke S, Yang J; Schizophrenia Working Group of the Psychiatric Genomics Consortium, Patterson N, Daly MJ, Price AL, Neale BM. LD Score regression distinguishes confounding from polygenicity in genome-wide association studies. Nat Genet. 2015 Mar;47(3):291-5. [PMID: 25642630]
32. Gaugler T, Klei L, Sanders SJ, Bodea CA, Goldberg AP, Lee AB, Mahajan M, Manaa D, Pawitan Y, Reichert J, Ripke S, Sandin S, Sklar P, Svantesson O, Reichenberg A, Hultman CM, Devlin B, Roeder K, Buxbaum JD. Most genetic risk for autism resides with common variation. Nat Genet. 2014 Aug;46(8):881-5. [PMID: 25038753]
33. Stein JL, Parikshak NN, Geschwind DH. Rare inherited variation in autism: beginning to see the forest and a few trees. Neuron. 2013 Jan 23;77(2):209-11. [PMID: 23352155]
34. Autism and Developmental Disabilities Monitoring Network Surveillance Year 2008 Principal Investigators; Centers for Disease Control and Prevention. Prevalence of autism spectrum disorders–Autism and Developmental Disabilities Monitoring Network, 14 sites, United States, 2008. MMWR Surveill Summ. 2012 Mar 30;61(3):1-19. [PMID: 22456193]
35. Duvekot J, van der Ende J, Verhulst FC, Slappendel G, van Daalen E, Maras A, Greaves-Lord K. Factors influencing the probability of a diagnosis of autism spectrum disorder in girls versus boys. Autism. 2017 Aug;21(6):646-658. [PMID: 27940569]
36. Werling DM, Geschwind DH. Understanding sex bias in autism spectrum disorder. Proc Natl Acad Sci U S A. 2013 Mar 26;110(13):4868-9. [PMID: 23476067]
37. Van Wijngaarden-Cremers PJ, van Eeten E, Groen WB, Van Deurzen PA, Oosterling IJ, Van der Gaag RJ. Gender and age differences in the core triad of impairments in autism spectrum disorders: a systematic review and meta-analysis. J Autism Dev Disord. 2014 Mar;44(3):627-35. [PMID: 23989936]
38. Werling DM, Geschwind DH. Recurrence rates provide evidence for sex-differential, familial genetic liability for autism spectrum disorders in multiplex families and twins. Mol Autism. 2015 May 13;6:27. [PMID: 25973164]
39. Baron-Cohen S. The extreme male brain theory of autism. Trends Cogn Sci. 2002 Jun 1;6(6):248-254. [PMID: 12039606]
40. Baron-Cohen S, Knickmeyer RC, Belmonte MK. Sex differences in the brain: implications for explaining autism. Science. 2005 Nov 4;310(5749):819-23. [PMID: 16272115]
41. Robinson EB, Lichtenstein P, Anckarsäter H, Happé F, Ronald A. Examining and interpreting the female protective effect against autistic behavior. Proc Natl Acad Sci U S A. 2013 Mar 26;110(13):5258-62. [PMID: 23431162]
42. Jacquemont S, Coe BP, Hersch M, Duyzend MH, Krumm N, Bergmann S, Beckmann JS, Rosenfeld JA, Eichler EE. A higher mutational burden in females supports a "female protective model" in neurodevelopmental disorders. Am J Hum Genet. 2014 Mar 6;94(3):415-25. [PMID: 24581740]
43. Werling DM, Parikshak NN, Geschwind DH. Gene expression in human brain implicates sexually dimorphic pathways in autism spectrum disorders. Nat Commun. 2016 Feb 19;7:10717. [PMID: 26892004]
44. Cross-Disorder Group of the Psychiatric Genomics Consortium. Identification of risk loci with shared effects on five major psychiatric disorders: a genome-wide analysis. Lancet. 2013 Apr 20;381(9875):1371-9. [PMID: 23453885]
45. Cross-Disorder Group of the Psychiatric Genomics Consortium, Lee SH, Ripke S, Neale BM, Faraone SV, Purcell SM, Perlis RH, Mowry BJ, Thapar A, Goddard ME, Witte JS, Absher D, Agartz I, Akil H, Amin F, Andreassen OA, Anjorin A, Anney R, Anttila V, Arking DE, Asherson P, Azevedo MH, Backlund L, Badner JA, Bailey AJ, Banaschewski T, Barchas JD, Barnes MR, Barrett TB, Bass N, Battaglia A, Bauer M, Bayés M, Bellivier F, Bergen SE, Berrettini W, Betancur C, Bettecken T, Biederman J, Binder EB, Black DW, Blackwood DH, Bloss CS, Boehnke M, Boomsma DI, Breen G, Breuer R, Bruggeman R, Cormican P, Buccola NG, Buitelaar JK, Bunney WE, Buxbaum JD, Byerley WF, Byrne EM, Caesar S, Cahn W, Cantor RM, Casas M, Chakravarti A, Chambert K, Choudhury K, Cichon S, Cloninger CR, Collier DA, Cook EH, Coon H, Cormand B, Corvin A, Coryell WH, Craig DW, Craig IW, Crosbie J, Cuccaro ML, Curtis D, Czamara D, Datta S, Dawson G, Day R, De Geus EJ, Degenhardt F, Djurovic S, Donohoe GJ, Doyle AE, Duan J, Dudbridge F, Duketis E, Ebstein RP, Edenberg HJ, Elia J, Ennis S, Etain B, Fanous A, Farmer AE, Ferrier IN, Flickinger M, Fombonne E, Foroud T, Frank J, Franke B, Fraser C, Freedman R, Freimer NB, Freitag CM, Friedl M, Frisén L, Gallagher L, Gejman PV, Georgieva L, Gershon ES, Geschwind DH, Giegling I, Gill M, Gordon SD, Gordon-Smith K, Green EK, Greenwood TA, Grice DE, Gross M, Grozeva D, Guan W, Gurling H, De Haan L, Haines JL, Hakonarson H, Hallmayer J, Hamilton SP, Hamshere ML, Hansen TF, Hartmann AM, Hautzinger M, Heath AC, Henders AK, Herms S, Hickie IB, Hipolito M, Hoefels S, Holmans PA, Holsboer F, Hoogendijk WJ, Hottenga JJ, Hultman CM, Hus V, Ingason A, Ising M, Jamain S, Jones EG, Jones I, Jones L, Tzeng JY, Kähler AK, Kahn RS, Kandaswamy R, Keller MC, Kennedy JL, Kenny E, Kent L, Kim Y, Kirov GK, Klauck SM, Klei L, Knowles JA, Kohli MA, Koller DL, Konte B, Korszun A, Krabbendam L, Krasucki R, Kuntsi J, Kwan P, Landén M, Långström N, Lathrop M, Lawrence J, Lawson WB, Leboyer M, Ledbetter DH, Lee PH, Lencz T, Lesch KP, Levinson DF, Lewis CM, Li J, Lichtenstein P, Lieberman JA, Lin DY, Linszen DH, Liu C, Lohoff FW, Loo SK, Lord C, Lowe JK, Lucae S, MacIntyre DJ, Madden PA, Maestrini E, Magnusson PK, Mahon PB, Maier W, Malhotra AK, Mane SM, Martin CL, Martin NG, Mattheisen M, Matthews K, Mattingsdal M, McCarroll SA, McGhee KA, McGough JJ, McGrath PJ, McGuffin P, McInnis MG, McIntosh A, McKinney R, McLean AW, McMahon FJ, McMahon WM, McQuillin A, Medeiros H, Medland SE, Meier S, Melle I, Meng F, Meyer J, Middeldorp CM, Middleton L, Milanova V, Miranda A, Monaco AP, Montgomery GW, Moran JL, Moreno-De-Luca D, Morken G, Morris DW, Morrow EM, Moskvina V, Muglia P, Mühleisen TW, Muir WJ, Müller-Myhsok B, Murtha M, Myers RM, Myin-Germeys I, Neale MC, Nelson SF, Nievergelt CM, Nikolov I, Nimgaonkar V, Nolen WA, Nöthen MM, Nurnberger JI, Nwulia EA, Nyholt DR, O'Dushlaine C, Oades RD, Olincy A, Oliveira G, Olsen L, Ophoff RA, Osby U, Owen MJ, Palotie A, Parr JR, Paterson AD, Pato CN, Pato MT, Penninx BW, Pergadia ML, Pericak-Vance MA, Pickard BS, Pimm J, Piven J, Posthuma D, Potash JB, Poustka F, Propping P, Puri V, Quested DJ, Quinn EM, Ramos-Quiroga JA, Rasmussen HB, Raychaudhuri S, Rehnström K, Reif A, Ribasés M, Rice JP, Rietschel M, Roeder K, Roeyers H, Rossin L, Rothenberger A, Rouleau G, Ruderfer D, Rujescu D, Sanders AR, Sanders SJ, Santangelo SL, Sergeant JA, Schachar R, Schalling M, Schatzberg AF, Scheftner WA, Schellenberg GD, Scherer SW, Schork NJ, Schulze TG, Schumacher J, Schwarz M, Scolnick E, Scott LJ, Shi J, Shilling PD, Shyn SI, Silverman JM, Slager SL, Smalley SL, Smit JH, Smith EN, Sonuga-Barke EJ, St Clair D, State M, Steffens M, Steinhausen HC, Strauss JS, Strohmaier J, Stroup TS, Sutcliffe JS, Szatmari P, Szelinger S, Thirumalai S, Thompson RC, Todorov AA, Tozzi F, Treutlein J, Uhr M, van den Oord EJ, Van Grootheest G, Van Os J, Vicente AM, Vieland VJ, Vincent JB, Visscher PM, Walsh CA, Wassink TH, Watson SJ, Weissman MM, Werge T, Wienker TF, Wijsman EM, Willemsen G, Williams N, Willsey AJ, Witt SH, Xu W, Young AH, Yu TW, Zammit S, Zandi PP, Zhang P, Zitman FG, Zöllner S, Devlin B, Kelsoe JR, Sklar P, Daly MJ, O'Donovan MC, Craddock N, Sullivan PF, Smoller JW, Kendler KS, Wray NR; International Inflammatory Bowel Disease Genetics Consortium (IIBDGC). Genetic relationship between five psychiatric disorders estimated from genome-wide SNPs. Nat Genet. 2013 Sep;45(9):984-94. [PMID: 23933821]
46. Autism Spectrum Disorders Working Group of The Psychiatric Genomics Consortium. Meta-analysis of GWAS of over 16,000 individuals with autism spectrum disorder highlights a novel locus at 10q24.32 and a significant overlap with schizophrenia. Mol Autism. 2017 May 22;8:21. [PMID: 28540026]
47. Jokiranta-Olkoniemi E, Cheslack-Postava K, Sucksdorff D, Suominen A, Gyllenberg D, Chudal R, Leivonen S, Gissler M, Brown AS, Sourander A. Risk of psychiatric and neurodevelopmental disorders among siblings of probands with autism spectrum disorders. JAMA Psychiatry. 2016 Jun 1;73(6):622-9. [PMID: 27145529]
48. Gillberg C. Chromosomal disorders and autism. J Autism Dev Disord. 1998 Oct;28(5):415-25. [PMID: 9813777]
49. Kielinen M, Rantala H, Timonen E, Linna SL, Moilanen I. Associated medical disorders and disabilities in children with autistic disorder: a population-based study. Autism. 2004 Mar;8(1):49-60. [PMID: 15070547]
50. Fine SE, Weissman A, Gerdes M, Pinto-Martin J, Zackai EH, McDonald-McGinn DM, Emanuel BS. Autism spectrum disorders and symptoms in children with molecularly confirmed 22q11.2 deletion syndrome. J Autism Dev Disord. 2005 Aug;35(4):461-70. [PMID: 16134031]
51. Gillberg IC, Gillberg C, Ahlsén G. Autistic behaviour and attention deficits in tuberous sclerosis: a population-based study. Dev Med Child Neurol. 1994 Jan;36(1):50-6. [PMID: 8132114]
52. Clarke TK, Lupton MK, Fernandez-Pujals AM, Starr J, Davies G, Cox S, Pattie A, Liewald DC, Hall LS, MacIntyre DJ, Smith BH, Hocking LJ, Padmanabhan S, Thomson PA, Hayward C, Hansell NK, Montgomery GW, Medland SE, Martin NG, Wright MJ, Porteous DJ, Deary IJ, McIntosh AM. Common polygenic risk for autism spectrum disorder (ASD) is associated with cognitive ability in the general population. Mol Psychiatry. 2016 Mar;21(3):419-25. [PMID: 25754080]
53. Moreno-De-Luca D, Sanders SJ, Willsey AJ, Mulle JG, Lowe JK, Geschwind DH, State MW, Martin CL, Ledbetter DH. Using large clinical data sets to infer pathogenicity for rare copy number variants in autism cohorts. Mol Psychiatry. 2013 Oct;18(10):1090-5. [PMID: 23044707]
54. Geschwind DH. Advances in autism. Annu Rev Med. 2009;60:367-80. [PMID: 19630577]
55. Jeste SS, Geschwind DH. Disentangling the heterogeneity of autism spectrum disorder through genetic findings. Nat Rev Neurol. 2014 Feb;10(2): 74-81. [PMID: 24468882]
56. Laumonnier F, Bonnet-Brilhault F, Gomot M, Blanc R, David A, Moizard MP, Raynaud M, Ronce N, Lemonnier E, Calvas P, Laudier B, Chelly J, Fryns JP, Ropers HH, Hamel BC, Andres C, Barthélémy C, Moraine C, Briault S. X-linked mental retardation and autism are associated with a mutation in the NLGN4 gene, a member of the neuroligin family. Am J Hum Genet. 2004 Mar;74(3):552-7. [PMID: 14963808]
57. Bucan M, Abrahams BS, Wang K, Glessner JT, Herman EI, Sonnenblick LI, Alvarez Retuerto AI, Imielinski M, Hadley D, Bradfield JP, Kim C, Gidaya NB, Lindquist I, Hutman T, Sigman M, Kustanovich V, Lajonchere CM, Singleton A, Kim J, Wassink TH, McMahon WM, Owley T, Sweeney JA, Coon H, Nurnberger JI, Li M, Cantor RM, Minshew NJ, Sutcliffe JS, Cook EH, Dawson G, Buxbaum JD, Grant SF, Schellenberg GD, Geschwind DH, Hakonarson H. Genome-wide analyses of exonic copy number variants in a family-based study point to novel autism susceptibility genes. PLoS Genet. 2009 Jun;5(6):e1000536. [PMID: 19557195]
58. Hoeffding LK, Trabjerg BB, Olsen L, Mazin W, Sparsø T, Vangkilde A, Mortensen PB, Pedersen CB, Werge T. Risk of psychiatric disorders among individuals with the 22q11.2 deletion or duplication: a Danish nationwide, register-based study. JAMA Psychiatry. 2017 Mar 1;74(3):282-290. [PMID: 28114601]
59. Kim HG, Kishikawa S, Higgins AW, Seong IS, Donovan DJ, Shen Y, Lally E, Weiss LA, Najm J, Kutsche K, Descartes M, Holt L, Braddock S, Troxell R, Kaplan L, Volkmar F, Klin A, Tsatsanis K, Harris DJ, Noens I, Pauls DL, Daly MJ, MacDonald ME, Morton CC, Quade BJ, Gusella JF. Disruption of neurexin 1 associated with autism spectrum disorder. Am J Hum Genet. 2008 Jan;82(1):199-207. [PMID: 18179900]
60. Marshall CR, Noor A, Vincent JB, Lionel AC, Feuk L, Skaug J, Shago M, Moessner R, Pinto D, Ren Y, Thiruvahindrapduram B, Fiebig A, Schreiber S, Friedman J, Ketelaars CE, Vos YJ, Ficicioglu C, Kirkpatrick S, Nicolson R, Sloman L, Summers A, Gibbons CA, Teebi A, Chitayat D, Weksberg R, Thompson A, Vardy C, Crosbie V, Luscombe S, Baatjes R, Zwaigenbaum L, Roberts W, Fernandez B, Szatmari P, Scherer SW. Structural variation of chromosomes in autism spectrum disorder. Am J Hum Genet. 2008 Feb;82(2):477-88. [PMID: 18252227]
61. Rujescu D, Ingason A, Cichon S, Pietiläinen OP, Barnes MR, Toulopoulou T, Picchioni M, Vassos E, Ettinger U, Bramon E, Murray R, Ruggeri M, Tosato S, Bonetto C, Steinberg S, Sigurdsson E, Sigmundsson T, Petursson H, Gylfason A, Olason PI, Hardarsson G, Jonsdottir GA, Gustafsson O, Fossdal R, Giegling I, Möller HJ, Hartmann AM, Hoffmann P, Crombie C, Fraser G, Walker N, Lonnqvist J, Suvisaari J, Tuulio-Henriksson A, Djurovic S, Melle I, Andreassen OA, Hansen T, Werge T, Kiemeney LA, Franke B, Veltman J, Buizer-Voskamp JE; GROUP Investigators, Sabatti C, Ophoff RA, Rietschel M, Nöthen MM, Stefansson K, Peltonen L, St Clair D, Stefansson H, Collier DA. Disruption of the neurexin 1 gene is associated with schizophrenia. Hum Mol Genet. 2009 Mar 1;18(5):988-96. [PMID: 18945720]
62. Stessman HA, Xiong B, Coe BP, Wang T, Hoekzema K, Fenckova M, Kvarnung M, Gerdts J, Trinh S, Cosemans N, Vives L, Lin J, Turner TN, Santen G, Ruivenkamp C, Kriek M, van Haeringen A, Aten E, Friend K, Liebelt J, Barnett C, Haan E, Shaw M, Gecz J, Anderlid BM, Nordgren A, Lindstrand A, Schwartz C, Kooy RF, Vandeweyer G, Helsmoortel C, Romano C, Alberti A, Vinci M, Avola E, Giusto S, Courchesne E, Pramparo T, Pierce K, Nalabolu S, Amaral DG, Scheffer IE, Delatycki MB, Lockhart PJ, Hormozdiari F, Harich B, Castells-Nobau A, Xia K, Peeters H, Nordenskjöld M, Schenck A, Bernier RA, Eichler EE. Targeted sequencing identifies 91 neurodevelopmental-disorder risk genes with autism and developmental-disability biases. Nat Genet. 2017 Apr;49(4):515-526. [PMID: 28191889]
63. Parikshak NN, Luo R, Zhang A, Won H, Lowe JK, Chandran V, Horvath S, Geschwind DH. Integrative functional genomic analyses implicate specific molecular pathways and circuits in autism. Cell. 2013 Nov 21;155(5):1008-21. [PMID: 24267887]
64. Schaefer GB, Mendelsohn NJ; Professional Practice and Guidelines Committee. Clinical genetics evaluation in identifying the etiology of autism spectrum disorders. Genet Med. 2008 Apr;10(4): 301-5. [PMID: 18414214]
65. Schaefer GB, Mendelsohn NJ; Professional Practice and Guidelines Committee. Clinical genetics evaluation in identifying the etiology of autism spectrum disorders: 2013 guideline revisions. Genet Med. 2013 May;15(5):399-407. [PMID: 23519317]
66. Fogel BL, Lee H, Strom SP, Deignan JL, Nelson SF. Clinical exome sequencing in neurogenetic and neuropsychiatric disorders. Ann N Y Acad Sci. 2016 Feb;1366(1):49-60. [PMID: 26250888]
67. Lee H, Deignan JL, Dorrani N, Strom SP, Kantarci S, Quintero-Rivera F, Das K, Toy T, Harry B, Yourshaw M, Fox M, Fogel BL, Martinez-Agosto JA, Wong DA, Chang VY, Shieh PB, Palmer CG, Dipple KM, Grody WW, Vilain E, Nelson SF. Clinical exome sequencing for genetic identification of rare Mendelian disorders. JAMA. 2014 Nov 12;312(18):1880-7. [PMID: 25326637]
68. Reiff M, Bugos E, Giarelli E, Bernhardt BA, Spinner NB, Sankar PL, Mulchandani S. "Set in stone" or "ray of hope": parents' beliefs about cause and prognosis after genomic testing of children diagnosed with ASD. J Autism Dev Disord. 2017 May;47(5):1453- 1463. [PMID: 28229350]
69. Xu L, Mitchell LC, Richman AR, Clawson K. What do parents think about chromosomal microarray testing? a qualitative report from parents of children with autism spectrum disorders. Autism Res Treat. 2016;2016:6852539. [PMID: 27413549]
70. Chess S. Autism in children with congenital rubella. J Autism Child Schizophr. 1971 Jan-Mar;1(1):33-47. [PMID: 5172438]
71. Chess S. Follow-up report on autism in congenital rubella. J Autism Child Schizophr. 1977 Mar;7(1):69-81. [PMID: 576606]
72. Strömland K, Nordin V, Miller M, Akerström B, Gillberg C. Autism in thalidomide embryopathy: a population study. Dev Med Child Neurol. 1994 Apr;36(4):351-6. [PMID: 8157157]
73. Willsey AJ, Sanders SJ, Li M, Dong S, Tebbenkamp AT, Muhle RA, Reilly SK, Lin L, Fertuzinhos S, Miller JA, Murtha MT, Bichsel C, Niu W, Cotney J, Ercan-Sencicek AG, Gockley J, Gupta AR, Han W, He X, Hoffman EJ, Klei L, Lei J, Liu W, Liu L, Lu C, Xu X, Zhu Y, Mane SM, Lein ES, Wei L, Noonan JP, Roeder K, Devlin B, Sestan N, State MW. Coexpression networks implicate human midfetal deep cortical projection neurons in the pathogenesis of autism. Cell. 2013 Nov 21;155(5):997-1007. [PMID: 24267886]
74. Stoner R, Chow ML, Boyle MP, Sunkin SM, Mouton PR, Roy S, Wynshaw-Boris A, Colamarino SA, Lein ES, Courchesne E. Patches of disorganization in the neocortex of children with autism. N Engl J Med. 2014 Mar 27;370(13):1209-1219. [PMID: 24670167]
75. Voineagu I, Wang X, Johnston P, Lowe JK, Tian Y, Horvath S, Mill J, Cantor RM, Blencowe BJ, Geschwind DH. Transcriptomic analysis of autistic brain reveals convergent molecular pathology. Nature. 2011 May 25;474(7351):380-4. [PMID: 21614001]
76. Atladóttir HO, Thorsen P, Østergaard L, Schendel DE, Lemcke S, Abdallah M, Parner ET. Maternal infection requiring hospitalization during pregnancy and autism spectrum disorders. J Autism Dev Disord. 2010 Dec;40(12):1423-30. [PMID: 20414802]
77. Schmidt RJ, Lyall K, Hertz-Picciotto I. Environment and autism: current state of the science. Cut Edge Psychiatry Pract. 2014 Summer;1(4):21-38. [PMID: 27453776]
78. Rodier PM, Ingram JL, Tisdale B, Croog VJ. Linking etiologies in humans and animal models: studies of autism. Reprod. Toxicol. 1997 Jun; 11(2-3):417–422. [PMID: 9100317]
79. Miyazaki K, Narita N, Narita M. Maternal administration of thalidomide or valproic acid causes abnormal serotonergic neurons in the offspring: implication for pathogenesis of autism. Int. J. Dev. Neurosci. Off. J. Int. Soc. Dev. Neurosci. 2005 May; 23(2-3):287–297. [PMID: 15749253]
80. Christensen J, Grønborg TK, Sørensen MJ, Schendel D, Parner ET, Pedersen LH, Vestergaard M. Prenatal valproate exposure and risk of autism spectrum disorders and childhood autism. JAMA. 2013 Apr; 309(16):1696. [PMID: 23613074]
81. Johnson S, Hollis C, Kochhar P, Hennessy E, Wolke D Marlow N. Psychiatric disorders in extremely preterm children: longitudinal finding at age 11 years in the EPICure study. J. Am. Acad. Child Adolesc. Psychiatry. 2010 May; 49(5):453–463.e1. [PMID: 20431465]
82. Limperopoulos C, Bassan H, Sullivan NR, Soul JS, Roberston RL Jr, Moore M, Ringer SA, Volpe JJ, du Plessis AJ. Positive screening for autism in ex-preterm infants: prevalence and risk factors. Pediatrics. 2008 Apr; 121(4):758–765. [PMID: 18381541]
83. Pinto-Martin JA, Levy SE, Feldman JF, Lorenz JM, Paneth N, Whitaker AH. Prevalence of autism spectrum disorder in adolescents born weighing <2000 grams. Pediatrics. 2011 Oct; 128(5):883–891. [PMID: 22007018]
84. Schieve LA, Rice C, Devine O, Maenner MJ, Lee LC, Fitzgerald R, Wingate MS, Schendel D, Pettygrove S, van Naarden Braun K, Durkin M. Have secular changes in perinatal risk factors contributed to the recent autism prevalence increase? development and application of a mathematical assessment model. Ann. Epidemiol. 2011 Dec; 21(12):930–945. [PMID: 22000328]
85. Sandin S, Hultman CM, Kolevzon A, Gross R, MacCabe JH, Reichenberg A. Advancing maternal age is associated with increasing risk for autism: a review and meta-analysis. J. Am. Acad. Child Adolesc. Psychiatry. 2012 May; 51(5):477–486.e1. [PMID: 22525954]
86. Hultman CM, Sandin S, Levine SZ, Lichtenstein P, Reichenberg A. Advancing paternal age and risk of autism: new evidence from a population-based study and a meta-analysis of epidemiological studies. Mol. Psychiatry. 2011 Dec; 16(12):1203–1212. [PMID: 21116277]
87. Puleo CM, Schmeidler J, Reichenberg A, Kolevzon A, Soorya LV, Buxbaum JD, Silverman JM. Advancing paternal age and simplex autism. Autism. 2012 Jul; 16(4):367–380. [PMID: 22180389]
88. Reichenberg A, Gross R, Sandin S, Susser ES. Advancing paternal and maternal age are both important for autism risk. Am. J. Public Health. 2010 May; 100(5):772–773; author reply 773. [PMID: 20299637]
89. Cheslack-Postava K, Liu K, Bearman PS. Closely spaced pregnancies are associated with increased odds of autism in California sibling births. Pediatrics. 2011 Feb; 127(2):246–253. [PMID: 21220394]
90. Gunnes N, Suren P, Bresnahan M, Hornig M, Lie KK, Lipkin WI, Magnus P, Nilsen RM, Reichborn-Kjennerud T, Scholberg S, Susser ES, Oyen AS, Stoltenberg C. Interpregnancy interval and risk of autistic disorder. Epidemiology. 2013 Nov; 24(6):906–912. [PMID: 24045716]
91. Surén P, Susser E, Stoltenberg C. Maternal folic acid supplementation and risk of autism–reply. JAMA. 2013 Jun 5;309(21):2208. [PMID: 23736721]
92. Schmidt RJ, Tancredi DJ, Ozonoff S, Hansen RL, Hartiala J, Allayee H, Schmidt LC, Tassone F, Hertz-Picciotto I. Maternal periconceptional folic acid intake and risk of autism spectrum disorders and developmental delay in the CHARGE (CHildhood Autism Risks from Genetics and Environment) case-control study. Am J Clin Nutr. 2012 Jul;96(1):80-9. [PMID: 22648721]
93. Kalkbrenner AE, Windham GC, Serre ML, Akita Y, Wang X, Hoffman K, Thayer BP, Daniels JL. Particulate matter exposure, prenatal and postnatal windows of susceptibility, and autism spectrum disorders. Epidemiology. 2015 Jan;26(1):30-42. [PMID: 25286049]
94. Raz R, Roberts AL, Lyall K, Hart JE, Just AC, Laden F, Weisskopf MG. Autism spectrum disorder and particulate matter air pollution before, during, and after pregnancy: a nested case-control analysis within the Nurses' Health Study II Cohort. Environ Health Perspect. 2015 Mar;123(3):264-70. [PMID: 25522338]
95. Bennett D, Bellinger DC, Birnbaum LS, Bradman A, Chen A, Cory-Slechta DA, Engel SM, Fallin MD, Halladay A, Hauser R, Hertz-Picciotto I, Kwiatkowski CF, Lanphear BP, Marquez E, Marty M, McPartland J, Newschaffer CJ, Payne-Sturges D, Patisaul HB, Perera FP, Ritz B, Sass J, Schantz SL, Webster TF, Whyatt RM, Woodruff TJ, Zoeller RT, Anderko L, Campbell C, Conry JA, DeNicola N, Gould RM, Hirtz D, Huffling K, Landrigan PJ, Lavin A, Miller M, Mitchell MA, Rubin L, Schettler T, Tran HL, Acosta A, Brody C, Miller E, Miller P, Swanson M, Witherspoon NO; American College of Obstetricians and Gynecologists (ACOG); Child Neurology Society; Endocrine Society; International Neurotoxicology Association; International Society for Children’s Health and the Environment; International Society for Environmental Epidemiology; National Council of Asian Pacific Islander Physicians; National Hispanic Medical Association; National Medical Association. Project TENDR: targeting environmental neuro-developmental risks the TENDR consensus statement. Environ Health Perspect. 2016 Jul 1;124(7):A118-22. [PMID: 27479987]
96. Lyall K, Croen L, Daniels J, Fallin MD, Ladd-Acosta C, Lee BK, Park BY, Snyder NW, Schendel D, Volk H, Windham GC, Newschaffer C. The changing epidemiology of autism spectrum disorders. Annu Rev Public Health. 2017 Mar 20;38:81-102. [PMID: 28068486]
97. Gee GC, Payne-Sturges DC. Environmental health disparities: a framework integrating psychosocial and environmental concepts. Environ Health Perspect. 2004 Dec;112(17):1645-53. [PMID: 15579407]
98. Evans GW, Kantrowitz E. Socioeconomic status and health: the potential role of environmental risk exposure. Annu Rev Public Health. 2002;23:303-31. [PMID: 11910065]
99. Adamkiewicz G, Zota AR, Fabian MP, Chahine T, Julien R, Spengler JD, Levy JI. Moving environmental justice indoors: understanding structural influences on residential exposure patterns in low-income communities. Am J Public Health. 2011 Dec;101 Suppl 1:S238-45. [PMID: 21836112]
100. Christensen DL, Bilder DA, Zahorodny W, Pettygrove S, Durkin MS, Fitzgerald RT, Rice C, Kurzius-Spencer M, Baio J, Yeargin-Allsopp M. Prevalence and characteristics of autism spectrum disorder among 4-year-old children in the Autism and Developmental Disabilities Monitoring Network. J Dev Behav Pediatr. 2016 Jan;37(1):1-8. [PMID: 26651088]
101. Cui Y, Balshaw DM, Kwok RK, Thompson CL, Collman GW, Birnbaum LS. The exposome: embracing the complexity for discovery in environmental health. Environ Health Perspect. 2016 Aug 1;124(8):A137-40. [PMID: 27479988]
102. Stingone JA, Buck Louis GM, Nakayama SF, Vermeulen RC, Kwok RK, Cui Y, Balshaw DM, Teitelbaum SL. Toward greater implementation of the exposome research paradigm within environmental epidemiology. Annu Rev Public Health. 2017 Mar 20;38:315-327. [PMID: 28125387]
103. Wild CP. The exposome: from concept to utility. Int J Epidemiol. 2012 Feb;41(1):24-32. [PMID: 22296988]
104. Rappaport SM. Implications of the exposome for exposure science. J Expo Sci Environ Epidemiol. 2011 Jan-Feb;21(1):5-9. [PMID: 21081972]
105. Ladd-Acosta C, Shu C, Lee BK, Gidaya N, Singer A, Schieve LA, Schendel DE, Jones N, Daniels JL, Windham GC, Newschaffer CJ, Croen LA, Feinberg AP, Daniele Fallin M. Presence of an epigenetic signature of prenatal cigarette smoke exposure in childhood. Environ Res. 2016 Jan;144(Pt A):139-148. [PMID: 26610292]
106. Houten SM, Chen J, Belpoggi F, Manservisi F, Sánchez-Guijo A, Wudy SA, Teitelbaum SL. Changes in the metabolome in response to low-dose exposure to environmental chemicals used in personal care products during different windows of susceptibility. PLoS One. 2016 Jul 28;11(7):e0159919. [PMID: 27467775]
107. Marsillach J, Costa LG, Furlong CE. Paraoxonase-1 and early-life environmental exposures. Ann Glob Health. 2016 Jan-Feb;82(1):100-10. [PMID: 27325068]
108. Mazina V, Gerdts J, Trinh S, Ankenman K, Ward T, Dennis MY, Girirajan S, Eichler EE, Bernier R. Epigenetics of autism-related impairment: copy number variation and maternal infection. J Dev Behav Pediatr. 2015 Feb-Mar;36(2):61-7. [PMID: 25629966]
109. Schmidt RJ, Hansen RL, Hartiala J, Allayee H, Schmidt LC, Tancredi DJ, Tassone F, Hertz-Picciotto I. Prenatal vitamins, one-carbon metabolism gene variants, and risk for autism. Epidemiology. 2011 Jul;22(4):476-85. [PMID: 21610500]
110. Volk HE, Kerin T, Lurmann F, Hertz-Picciotto I, McConnell R, Campbell DB. Autism spectrum disorder: interaction of air pollution with the MET receptor tyrosine kinase gene. Epidemiology. 2014 Jan;25(1):44-7. [PMID: 24240654]
111. Webb SJ, Garrison MM, Bernier R, McClintic AM, King BH, Mourad PD. Severity of ASD symptoms and their correlation with the presence of copy number variations and exposure to first trimester ultrasound. Autism Res. 2017 Mar;10(3):472-484. [PMID: 27582229]
112. Gauderman WJ, Mukherjee B, Aschard H, Hsu L, Lewinger JP, Patel CJ, Witte JS, Amos C, Tai CG, Conti D, Torgerson DG, Lee S, Chatterjee N. Update on the state of the science for analytical methods for gene-environment interactions. Am J Epidemiol. 2017 Oct 1;186(7):762-770. [PMID: 28978192]
113. Kim D, Volk H, Girirajan S, Pendergrass S, Hall MA, Verma SS, Schmidt RJ, Hansen RL, Ghosh D, Ludena- Rodriguez Y, Kim K, Ritchie MD, Hertz-Picciotto I, Selleck SB. The joint effect of air pollution exposure and copy number variation on risk for autism. Autism Res. 2017 Apr 27. [PMID: 28448694]
114. Pearson BL, Simon JM, McCoy ES, Salazar G, Fragola G, Zylka MJ. Identification of chemicals that mimic transcriptional changes associated with autism, brain aging and neurodegeneration. Nat Commun. 2016 Mar 31;7:11173. [PMID: 27029645]
115. Ben-Reuven L, Reiner O. Modeling the autistic cell: iPSCs recapitulate developmental principles of syndromic and nonsyndromic ASD. Dev Growth Differ. 2016 Jun;58(5):481-91. [PMID: 27111774]
116. Hogberg HT, Bressler J, Christian KM, Harris G, Makri G, O'Driscoll C, Pamies D, Smirnova L, Wen Z, Hartung T. Toward a 3D model of human brain development for studying gene/environment interactions. Stem Cell Res Ther. 2013;4 Suppl 1:S4. [PMID: 24564953]
117. Carter CJ, Blizard RA. Autism genes are selectively targeted by environmental pollutants including pesticides, heavy metals, bisphenol A, phthalates and many others in food, cosmetics or household products. Neurochem Int. 2016 Oct 27. [PMID: 27984170]
118. Chesler EJ. Out of the bottleneck: the Diversity Outcross and Collaborative Cross mouse populations in behavioral genetics research. Mamm Genome. 2014 Feb;25(1-2):3-11. [PMID: 24272351]
119. Ladd-Acosta C, Fallin MD. The role of epigenetics in genetic and environmental epidemiology. Epigenomics. 2016 Feb;8(2):271-83. [PMID: 26505319]
120. Keil KP, Lein PJ. DNA methylation: a mechanism linking environmental chemical exposures to risk of autism spectrum disorders? Environ Epigenet. 2016 Mar;2(1). pii: dvv012. [PMID: 27158529]
121. Vogel Ciernia A, LaSalle J. The landscape of DNA methylation amid a perfect storm of autism aetiologies. Nat Rev Neurosci. 2016 Jul;17(7):411-23. [PMID: 27150399]
122. Schroeder DI, Schmidt RJ, Crary-Dooley FK, Walker CK, Ozonoff S, Tancredi DJ, Hertz-Picciotto I, LaSalle JM. Placental methylome analysis from a prospective autism study. Mol Autism. 2016 Dec 15;7:51. [PMID: 28018572]
123. Feinberg JI, Bakulski KM, Jaffe AE, Tryggvadottir R, Brown SC, Goldman LR, Croen LA, Hertz-Picciotto I, Newschaffer CJ, Fallin MD, Feinberg AP. Paternal sperm DNA methylation associated with early signs of autism risk in an autism-enriched cohort. Int J Epidemiol. 2015 Aug;44(4):1199-210. [PMID: 25878217]
124. Hou L, Zhang X, Wang D, Baccarelli A. Environmental chemical exposures and human epigenetics. Int J Epidemiol. 2012 Feb;41(1):79-105. [PMID: 22253299]
125. Feil R, Fraga MF. Epigenetics and the environment: emerging patterns and implications. Nat Rev Genet. 2012 Jan 4;13(2):97-109. [PMID: 22215131]
126. Bakulski KM, Fallin MD. Epigenetic epidemiology: promises for public health research. Environ Mol Mutagen. 2014 Apr;55(3):171-83. [PMID: 24449392]
127. Bakulski KM, Halladay A, Hu VW, Mill J, Fallin MD. Epigenetic research in neuropsychiatric disorders: the "tissue issue". Curr Behav Neurosci Rep. 2016 Sep;3(3):264-274. [PMID: 28093577]
128. Meltzer A, Van de Water J. The role of the immune system in autism spectrum disorder. Neuropsychopharmacology. 2017 Jan;42(1): 284-298. [PMID: 27534269]
129. Tylee DS, Hess JL, Quinn TP, Barve R, Huang H, Zhang-James Y, Chang J, Stamova BS, Sharp FR, Hertz-Picciotto I, Faraone SV, Kong SW, Glatt SJ. Blood transcriptomic comparison of individuals with and without autism spectrum disorder: A combined-samples mega-analysis. Am J Med Genet B Neuropsychiatr Genet. 2017 Apr;174(3):181- 201. [PMID: 27862943]
130. Krakowiak P, Goines PE, Tancredi DJ, Ashwood P, Hansen RL, Hertz-Picciotto I, Van de Water J. Neonatal cytokine profiles associated with autism spectrum disorder. Biol Psychiatry. 2017 Mar 1;81(5):442-451. [PMID: 26392128]
131. Schug TT, Blawas AM, Gray K, Heindel JJ, Lawler CP. Elucidating the links between endocrine disruptors and neurodevelopment. Endocrinology. 2015 Jun;156(6):1941-51. [PMID: 25714811]
132. Park BY, Lee BK, Burstyn I, Tabb LP, Keelan JA, Whitehouse AJO, Croen LA, Fallin MD, Hertz-Picciotto I, Montgomery O, Newschaffer CJ. Umbilical cord blood androgen levels and ASD-related phenotypes at 12 and 36 months in an enriched risk cohort study. Mol Autism. 2017 Jan 31;8:3. [PMID: 28163867]
133. Fung TC, Olson CA, Hsiao EY. Interactions between the microbiota, immune and nervous systems in health and disease. Nat Neurosci. 2017 Feb;20(2): 145-155. [PMID: 28092661]
134. Vuong HE, Hsiao EY. Emerging roles for the gut microbiome in autism spectrum disorder. Biol Psychiatry. 2017 Mar 1;81(5):411-423. [PMID: 27773355]
135. Gao B, Chi L, Mahbub R, Bian X, Tu P, Ru H, Lu K. Multi-omics reveals that lead exposure disturbs gut microbiome development, key metabolites, and metabolic pathways. Chem Res Toxicol. 2017 Apr 17;30(4):996-1005. [PMID: 28234468]
136. Lu K, Abo RP, Schlieper KA, Graffam ME, Levine S, Wishnok JS, Swenberg JA, Tannenbaum SR, Fox JG. Arsenic exposure perturbs the gut microbiome and its metabolic profile in mice: an integrated metagenomics and metabolomics analysis. Environ Health Perspect. 2014 Mar;122(3):284-91. [PMID: 24413286]
137. Alava P, Du Laing G, Tack F, De Ryck T, Van De Wiele T. Westernized diets lower arsenic gastrointestinal bioaccessibility but increase microbial arsenic speciation changes in the colon. Chemosphere. 2015 Jan;119:757-62. [PMID: 25192650]
138. D C Rubin SS, Alava P, Zekker I, Du Laing G, Van de Wiele T. Arsenic thiolation and the role of sulfate-reducing bacteria from the human intestinal tract. Environ Health Perspect. 2014 Aug;122(8): 817-22. [PMID: 24833621]
139. Mattingly CJ, Boyles R, Lawler CP, Haugen AC, Dearry A, Haendel M. Laying a community-based foundation for data-driven semantic standards in environmental health sciences. Environ Health Perspect. 2016 Aug;124(8):1136-40. [PMID: 26871594]
140. Hamilton CM, Strader LC, Pratt JG, Maiese D, Hendershot T, Kwok RK, Hammond JA, Huggins W, Jackman D, Pan H, Nettles DS, Beaty TH, Farrer LA, Kraft P, Marazita ML, Ordovas JM, Pato CN, Spitz MR, Wagener D, Williams M, Junkins HA, Harlan WR, Ramos EM, Haines J. The PhenX Toolkit: get the most from your measures. Am J Epidemiol. 2011 Aug 1;174(3):253-60. [PMID: 21749974]